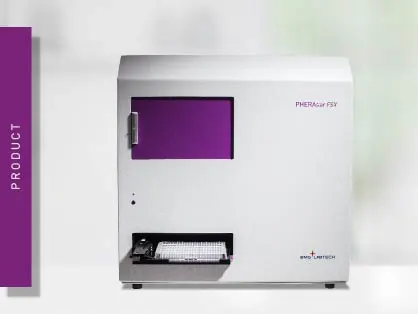
PHERAstar FSX
Powerful and most sensitive HTS plate reader
G protein-coupled receptors are a gateway to many cellular responses. Find out how microplate readers can be used to support GPCR research.
G protein-coupled receptors (GPCRs) are highly sophisticated detection molecules that can respond to signals as diverse as a photon or a hormone. They are as old as the first multicellular organisms that appeared on the Earth around 600 million years ago. And if you need to take an FDA-approved drug, there’s around a one in three chance that it’s a drug that targets a GPCR. Collectively, the GPCR family represents the largest family of proteins and includes at least 1000 GPCRs in humans. But what are the best ways to study this diverse group of molecules and the cascade of signaling events that ensue in the cell?
In the first part of this blog article, we look at what GPCRs are, some of their responsibilities in the cell, and the overall approaches used to study these signaling molecules. In the second part, we take a deeper dive into how microplate-based assays are used to support GPCR research, how detection technologies are deployed for specific applications, and give some examples of solutions developed for interaction assays, reporter assays, or immunoassays. The goal is to give a sense of the scale and scope of how microplate readers can be used to study GPCR signaling as well as the potential for future applications in this rapidly growing area of research with many unmet needs.
GPCRs contribute to many important functions in the human body and play a crucial role in health and disease. They serve as entry points into the cell for external signals, which include hormones, neurotransmitters, therapeutics and potential drugs like cannabinoids, and other environmental stimuli. GPCRs are also essential for vision, smell, and taste (sensory GPCRs), bridging input signals to a cascade of signaling pathways within the cell.
Since they are so widespread and an integral part of many cell signaling pathways, GPCRs are popular targets for drug screening and development. More than 30% of FDA-approved drugs target GPCRs.1 Of the 360 non-sensory GPCRs, only around 36% have been targeted by drugs which leaves more than 200 non-sensory GPCRs as new targets to be explored for drug development. 1 Sensory and non-sensory GPCRs are therefore promising avenues for further research.
GPCRs are seven-pass transmembrane domain receptors (fig.1). The N-terminus of the receptor resides on the outside of the cell and is followed in turn by seven α-helices that pass through the cell membrane. The end of the receptor or C-terminus sits within the cell. The extracellular part of the receptor binds the ligand or stimulus. The intracellular C-terminus is crucial for interacting with GTP-binding proteins or G-proteins and sets in motion the signaling cascade within the cell.
Inside the cell, the G-proteins serve as intracellular molecular switches. Heterotrimeric G-proteins, which consist of three subunits (Gα, Gβ, and Gγ), are the class of G-proteins that interact with GPCRs, and they are typically bound to the receptor. They pass on the signal from the activated GPCRs to the cell interior and lead to a cascade of interactions and enzyme activities that ultimately brings about a change in cell function.
The molecular diversity of G-proteins does not stop there. Different types of G-proteins, which vary in their alpha subunit (e.g. Gs, Gi and Gq), are present in GPCRs (fig.2; the letters in the schematic highlight steps in the signaling pathway that can be analysed by specific assays. An extensive list of all these assays can be found at GPCR application notes. These different types of alpha subunit each activate an enzyme that either increases or decreases a second messenger [for example cyclic adenosine monophosphate (cAMP) or inositol trisphosphate (IP3)]. The change in second messenger impacts further downstream effectors that ultimately lead to a cellular response. For example, the differential activation of G-proteins by different ligands was studied in this application note using a new Bioluminescence Resonance Energy Transfer (BRET) biosensor for cAMP. In another approach, the TRUPATH platform, employing BRET assays as a robust and complete way to measure G-protein signaling, was used.
BMG LABTECH Application Notes
Today, most research studies of GPCR signaling events involve cell-based approaches. This is mainly due to the inherent instability of purified GPCRs when they are outside the environment of the cell or membrane which makes in vitro studies challenging. Cell-based assays are therefore the method of choice for researchers including those interested in high-throughput assays for drug screening.
There is no one-fit universal GPCR signaling assay which means that researchers must choose the solution that works best for the signaling events they are interested in. Most of the solutions available to scientists fall within the three categories: interaction assays, reporter assays or immunoassays.
GPCR researchers are interested in looking at the ways signaling molecules bind and interact with GPCRs, the kinetics of these interactions (binding kinetics), working out which Gα protein subunit is coupled to a specific GPCR, looking at how some ligands selectively activate some signaling pathways while blocking others (GPCR-biased agonism), or revealing the many downstream effects that ultimately lead to a functional response within a cell.
At one level, scientists, including those looking at high throughput assays, need to measure changes in the concentrations of second messengers that arise after activation of the membrane receptor. Many of these assays involve looking at shifts in the levels of cyclic adenosine monophosphate (cAMP) or calcium.
Signal transduction from active GPCRs must also eventually be turned off. Arrestins are a small family of proteins whose task is to turn off or desensitize activated GPCRs when they are recruited by the active phosphorylated receptors. β-arrestin recruitment-based assays are often used as reporters for GPCR activation and are a very useful tool for cell-based drug discovery efforts. Find out why BMG LABTECH microplate readers are ideally suited for these purposes in our dedicated GPCR brochure.
Structural characterization of GPCRs
For many years, crystal structures of GPCRs were difficult to obtain which hindered investigations into receptor function. The first crystal structure of a rhodopsin was reported in 2000 (fig.3).2 The molecular structure and function of the human β2 adrenergic GPCR followed in 2007.3 Two years later the crystal structure of a GPCR with a bound ligand was published.4 To date, crystallization and structural characterization of GPCRs requires protein engineering to stabilize the membrane proteins. Methods to stabilize GPCRs through point mutations have therefore been developed which are also useful ways to probe the relationship between structure and physiological function.5
Researchers can use fluorescently labelled ligands such as GFP for high-throughput stability tests of mutant receptors. Alternatively, Time-Resolved Förster’s Resonance Energy Transfer (TR-FRET) is an attractive method to measure changes in protein stability and function in the presence of different ligands. Intrinsic tryptophan fluorescence has also been used to determine the thermostability of GPCRs and to perform stability studies in the presence of denaturants.5 Site-directed mutagenesis can also be used to study the effects of drugs on GPCRs. Novel approaches utilising red-shifted dyes to reduce autofluorescence and to allow multiplexing of samples are also emerging.
As mentioned earlier, most of the solutions available to scientists fall within the three categories: interaction assays, reporter assays or immunoassays.
G protein-coupled receptor interaction assays
Interaction assays involve a wide range of detection technologies to look at how different signaling molecules interact (fig. 4). This includes ways to look at how a membrane receptor interacts with its ligand or how a compound might interfere with this interaction. You can learn more about how microplate-based interaction assays are used for drug discovery in the following video from BMG LABTECH: Interaction assays.
Interaction assays are needed to not only look at ligand binding but to also investigate binding kinetics. The study of binding kinetics is crucial for drug screening and research and the interaction of a ligand with a receptor is not a static process. Experiments looking at binding kinetics are designed to investigate the interactions between two or more molecules over time. Changes in binding comprise association and dissociation events and information about how long it takes a ligand to associate or dissociate from a receptor is crucial to understanding the affinity of a ligand for a receptor. More information on how to determine binding affinities on microplates is provided in this video from BMG LABTECH:
In the case of a drug, binding affinities can reveal how long it might take for it to become active or how long it will remain active, which is crucial information to inform the drug discovery process. This application note on a high-throughput assay for kinase inhibitors gives an example of a TR-FRET-based binding kinetic study. How binding kinetics work and which approaches can be used to study them on microplate readers are covered in detail in the blog article Binding kinetics and also in this E-Book on binding kinetics from BMG LABTECH.
G protein-coupled receptor reporter assays
Reporter assays typically involve a reporter gene that encodes a range of reporter molecules for example Green Fluorescent Protein (GFP) or luciferase (fig.5). Reporter gene expression linked to transcriptional regulation can be used to study G-protein-related responses that are activated by GPCR ligands. Reporter genes are typically used to measure receptor-mediated cell responses at the transcriptional or translational levels. For example, the firefly luciferase gene responds rapidly to GPCR modulation by agonists or antagonists. Luciferase assays have been used for high-throughput screening applications in drug discovery as well as the study of deorphanized GPCRs.
For arrestin recruitment-based assays, different reporters are available and include luciferase, GFP, and split-luciferase complementary assays.1 In addition, researchers may want to measure cytokine levels or gene expression that respond to cell signaling events or changes in second messengers.
Other biosensors
In addition to gene reporter assays, a range of biosensors are available to measure the specific levels of crucial second messengers or other signaling molecules in the cell like Ca2+ or cAMP. Different reagent kits are commercially available for this purpose. Many of these assays are based on the use of molecules to bind cAMP in a way that allows measurement by different detection technologies. Specific dyes like Fluo-4 can be used to measure calcium levels.
Other options are available to detect the levels of inositol monophosphate and diacylglycerol, second messengers whose levels rise prior to increases in calcium.
The application note HitHunter® cAMP XS+ assay for GPCR screening using the PHERAstar FSX describes ways to measure changes in the intracellular concentrations of cAMP that depend on luminescence and use of a reporter. The HitHunter® cAMP XS+ assay from DiscoverX is particularly useful for screening challenging targets with low basal levels of cAMP or low levels of cAMP response. In the study, a cAMP assay based on the enzyme fragmentation complementation (EFC) of β-galactosidase was used (fig. 6). Changes in the intracellular cAMP levels correlated with GPCR activation and measurements were suitable for high-throughput screening.
Immunoassays
In addition to interaction and reporter assays, microplate-based immunoassays are a versatile tool to investigate GPCR function (fig. 7). The selection of the right immunoassay can provide increased sensitivity, reduced background noise and improved stability for detection of molecules of interest.Today researchers have many microplate reader and detection options at their fingertips. How can microplate readers support GPCR research and which features are beneficial for specific applications?
Here we first discuss which microplate reader features are relevant and can facilitate GPCR research. Next, we discuss detection modes commonly used for GPCR assay quantification and give specific examples of where researchers have developed solutions for their interaction assays, reporter assays or immunoassays (fig. 2). Finally, we take a brief look at past and future research on GPCRs. These solutions support discovery-based research at any scale all the way up to the needs of high-throughput drug screening.
Bottom reading
As mentioned earlier, most assays for GPCRs are cell-based. In a plate reader, the bottom reading capabilities provide robust measurements for cell-based assays since the distance between the cell layer and detector is reduced. This minimizes unwanted effects including those from the cell culture medium. Microplate readers offering a software-controlled top/bottom detection switch provide flexibility and avoid the need for manual intervention, which is especially important for automated solutions.
Atmospheric Control
When running cell-based kinetic assays, a physiological environment is beneficial and effectively avoids biased responses caused by stressed cells. For this purpose, modern microplate readers can be equipped with gas and temperature control units. BMG LABTECH´s Atmospheric Control Unit (ACU) provides an optimal environment for real-time cell-based assays where stable O2 and CO2 tensions can be maintained and user-defined protocols for O2 deprivation and reoxygenation are possible.
Sampling rate
As cell signaling and protein interaction can be very fast events, a fast detection by the microplate reader is paramount. A high sampling rate is extremely beneficial as it enables the acquisition of fast events with no data loss or a higher data density within a set detection time frame. For example, the CLARIOstar® Plus can measure rapid changes of up to 100 data points per second in fluorescence intensity of Fluo-4, a cytoplasmic Ca2+ indicator used to measure transient changes in this second messenger (fig. 8). The use of reagent injectors that allow for simultaneous dispensing and data measurement combined with a fast sampling rate enables high signal resolution and fast measurement responses.If you are relying on TR-FRET interaction assays to analyse kinetic binding, the PHERAstar® FSX plate reader can be equipped with a TRF laser. Besides providing wavelength-specific high energy excitation, the TRF laser allows for faster detection (60 Hertz flash frequency) at high sensitivity and with optimal statistical performance.
High-throughput capability
As GPCRs are an integral part of many cell signaling pathways, they are a major target for drug discovery and screening. Accordingly, the ability to establish, run and evaluate assays at high throughput is crucial. Microplate readers like the PHERAstar® FSX are essential for this purpose since they are capable of high sensitivity and fast detection times. Moreover, the ability to read high-density microplate formats such as 384-, 1536- or even 3456-well microplates means low sample volumes translate into cost-effective solutions.
Simultaneous Dual Emission (SDE) enables accuracy and speed for measurements of samples in high-density plate formats. Detection technologies like TR-FRET, AlphaPLEX and BRET, which are commonly used for GPCR studies, emit at two wavelengths. The ratio of the measurements at these two wavelengths is the result that is reported. Almost all microplate readers suitable for drug discovery and high-throughput screening have one—or often two—paired sets of photomultiplier detectors that simultaneously monitor both emission wavelengths from a FRET or BRET assay. SDE means that data can be collected in one detection pass rather than two, which halves read times and doubles the throughput. Both emission wavelengths are measured at the same time under the same conditions with SDE. If measurements are made sequentially at different times, the ratio between the emission wavelengths may be perturbed by reaction kinetics, bubbles, evaporation, vibration or noise from other sources. If SDE is used to make measurements at both wavelengths simultaneously, coefficient of variation and Z-prime values are improved because the conditions are identical for both measurements.
Cross-talk reduction
When running luminescence and AlphaScreen® assays in high-density microplate formats, cross-talk can negatively affect assay data quality and statistics, as it can artificially increase measurement counts depending on the signal from the surrounding wells (fig. 9). Solutions for cross-talk reduction have to be considered as they can improve data quality, especially in screening assays. Plate readers like the CLARIOstar Plus, VANTAstar®, and PHERAstar FSX come with hardware and software-based solutions for cross-talk reduction.Analysis of binding kinetics
Interaction assays often require measurements of kinetics. Even though binding kinetics can be analysed by surface plasmon resonance or biolayer interferometry, microplate readers have established themselves as the gold standard. With the microplate format, binding kinetics can be miniaturised, enabling the high-throughput kinetic screening of large compound libraries.
In particular, the PHERAstar FSX offers unique and dedicated features to efficiently monitor kinetic binding events. These include high temporal resolution for the fastest kinetic events, reagent injection at point of read to not miss any data, and FRET, TR-FRET, Fluorescence Polarization and BRET kinetic detection.
The types of assays researchers perform for GPCRs mostly include interaction assays, reporter assays and immunoassays. What types of detection methods are the most suitable for these types of assays on microplates? For the most part fluorescence- or luminescence-based methods are the preferred choice and include the following:
Fluorescence Intensity (FI)
Fluorescence intensity measurements are mainly used in reporter assays and immunoassays. Under the right conditions, fluorescence intensity correlates linearly with the concentration of the molecule to be measured (e.g., analyte coupled to the excited fluorophore) which makes it appropriate for quantitative analyses.
An example of signaling that involves fluorescence intensity measurements, and a reporter assay is described in the application note Cellular dopamine and intracellular calcium signaling using the next generation HTS microplate reader. The method relies on use of the Fluo-4 Direct™ Calcium Assay from ThermoFisher Scientific to measure Ca2+ as a second messenger. Fluo-4 is a dye that if excited at a wavelength of 480 nm and shows an increase in fluorescence intensity at an emission wavelength of 520 nm if the levels of calcium ions have increased. Changes in calcium levels were measured in HEK 293 cells using a direct optic bottom reading of the samples in the microplate (fig.10). Bottom reading of the microplate provides better measurements since the distance between the cell layer and detector is reduced which minimizes unwanted effects from the cell culture medium.
FRET and TR-FRET
FRET and TR-FRET are often used to study molecular interactions or binding events. FRET and TR-FRET both depend on energy transfer between two fluorophores. In the case of TR-FRET, the donor fluorophore is a longer-lived lanthanide which results in significant improvements to background noise levels. TR-FRET offers significantly reduced background and an increased detection window between negative and positive signals compared to FRET (fig. 11). The lanthanide emission is more stable over time. As a homogeneous assay, detection of the donor-acceptor complex does not require physical separation from unbound components. This eliminates the need for separation steps as well as the multiple washing steps needed in other methods.
The application note HTRF® IP-One assay used for functional screenings describes how Homogeneous Time-Resolved Fluorescence (HTRF®) can be used together with a functional inositol phosphate 1 (IP1) assay for drug screening purposes (interaction assays). In this case, ligand binding is detected through a competitive immunoassay where free IP1 competes against IP1-d2 (the HTRF acceptor) for binding to an anti-IP1 cryptate conjugate (HTRF donor) (fig. 12).
IP1 is a stable downstream metabolite of IP3 which forms due to activation of a phospholipase C (PLC) cascade. The short half-life of IP3 makes it unsuitable for drug screening operations. However, IP1, a downstream metabolite of IP3, overcomes these limitations which makes it suitable for primary and secondary screening.The advantages of using TR-FRET include ease of use, its sensitivity, as well as its ability to scale through automation. The IP1 assay was shown to be a fast and reliable method to screen for many drug-like molecules that target protein-protein interactions on the PHERAstar FSX microplate reader. The application note describes how a robust assay was established using either fresh or frozen Chinese Hamster Ovary cells.
In the application note Analyze binding kinetics with HTRF®: determine kon and koff by association experiments on the PHERAstar, Cisbio’s Tag-lite® assay was used to measure the kinetics of Spiperone-d2 binding to the cellular dopamine D2 receptor on a PHERAstar FSX microplate reader. The dopamine receptor D is a GPCR that represents a target of action for antipsychotic drugs. Enzyme kinetic measurements were made to determine the koff and kon rates for the rate of Spiperone-d2 binding to the receptor (fig. 13).
Again, the advantages of using this type of homogeneous assay include avoiding the use of radioactive probes and the ability to scale up for automation and throughput due to the reduced number of steps needed for this type of assay. The homogeneous Tag-lite assay combined with the speed of the PHERAstar FSX microplate reader allowed for improved monitoring of the binding dynamics. The koff and kon rates could be used to calculate the dissociation constant KD of the receptor which provides useful information of the affinity of the drug for the GPCR target.
In the application note Cellular dopamine and intracellular calcium signaling using the next generation HTS microplate reader, the Tango™ Dopamine Receptor 1 assay was used to investigate arrestin recruitment by the dopamine D1 receptor (interaction assay) (fig. 14). Arrestin is responsible for turning off or desensitizing GPCRs. In a FRET-based assay, the inactivated receptor produces a green signal. After activation, the coumarin substrate is cleaved which leads to an increase in blue signal at 460 nm. The ratio of blue to green cells is an indicator of receptor activation and is a suitably robust assay for high-throughput screening. In both cell-based assays, direct optic bottom reading, dual emission detection and high resolution well scanning greatly enhanced the quality of the results arising from each assay. The arrestin application is a good example of how FRET can be used to investigate interaction between a specific membrane-bound GPCR and a specific protein, in this case an arrestin responsible for desensitizing the activity of the GPCR.
BRET
BRET is a ratiometric luminescent-type detection method that is mainly used in cell-based assays. The donor in BRET is a luciferase enzyme that generates emission light through an enzyme reaction. Luminescence excites an acceptor fluorophore if the donor and acceptor are near one another. NanoBRET™ offers a stronger signal and less background noise compared to classically used BRET components due to modifications made to a specific luciferase enzyme isolated from a species of deep-sea shrimp (fig. 15).
In the application note NanoBRET™ assay for monitoring of ligand binding to GPCRs in live cells, using the CLARIOstar® and the PHERAstar® FSX the donor luciferase NanoLuc is used in a nonradioactive, proximity-based interaction assay. This assay enables NanoBRET to be used for monitoring ligand binding to GPCRs in live cells. A GPCR was expressed in live HEK293 cells with NanoLuc luciferase at the N-terminus. The ligand of the receptor was labelled with a different fluorophore whose excitation spectrum overlapped the emission spectrum of NanoLuc. When the ligand is not bound to the receptor, only the blue emission of NanoLuc is detected. In contrast, if the ligand does bind to the receptor, red fluorescence is detected due to excitation of the fluorescence label by resonance energy transfer.
Compared to BRET, NanoBRET offers distinct advantages in the range and breadth of assays available as well as better performance due to improved spectral separation between donor and acceptor emission. NanoBRET offers an order of magnitude improvement in sensitivity over other existing BRET options. Some of the advantages of using NanoBRET over other bioluminescent assays and techniques like FRET, which include increased sensitivity, are discussed in detail in the BMG LABTECH blog article NanoBRET.
TruPATH is a BRET-based technology platform that enables discrete coupling of GPCR to G-proteins in a way that facilitates the discovery of drugs biased towards desired G protein effectors.6 In the scientific talk "Using the TruPATH BRET platform to analyse GPCR biased agonism", the design and optimization of TruPATH biosensor components, a protocol for its use, and examples of its potential for drug discovery through G protein bias are described.
TruPATH allows monitoring of 14 Renilla luciferase-fused Gα proteins with green fluorescent protein 2 (GFP2) Gγ subunits of their cognate GβγC heterodimers. A BRET signal is produced when Gα-RLuc is in close association with GFP2-Gγ as part of an intact heterotrimer. When the receptor is activated, the heterotrimer dissociates and the BRET signal decreases. The advantage of this approach is the specificity of the measurements towards specific G-proteins as part of the signaling process.
The first biosensors of heterotrimeric G proteins were designed for FRET. Later this approach was amended to take advantage of BRET. TruPATH expanded on the use of BRET in a way that is suitable for 96-well and 384-well formats. The Gαβγ biosensors of TruPATH, which were generated by protein engineering, interrogate the transducerome with single pathway resolution in cells. The TruPATH suite of Gαβγ biosensors includes the first Gα15 and GαGustducin probes. More information about using the TruPATH BRET platform to analyse GPCR-biased agonism is provided in this scientific presentation from Jeffrey Frederick DiBerto:
The ability to use NanoLuc for NanoBRET measurements is facilitated by powerful new gene editing technologies like CRISPR/Cas9. The application note CRISPR/Cas9 genome-edited cells express nanoBRET-donor that monitors protein interaction and trafficking describes the successful fusion of the NanoBRET donor to the GPCR CXCR4 using CRISPR/Cas9 gene editing. CRISPR/Cas9 enabled insertion of the DNA encoding NanoLuc into the endogenous genomic locus of CXCR4 in HEK293FT cells. Luminescence arising from the protein-luciferase fusion was sufficient to monitor receptor-protein interactions as well as protein trafficking (fig. 16). The studies were performed on the CLARIOstar using filters or the LVF Monochromator™.
Fluorescence polarization
Fluorescence polarization (FP) depends on measurement of the parallel and perpendicular emission intensities of plane polarized light. Polarized light arises when the direction of the electric field of light is restricted to one plane by a specific filter. In this case all selected light waves oscillate in a single direction. FP is widely used to study molecular interactions in solution, including protein-protein interactions, protein-DNA binding, enzyme activity and other molecular interactions.
In the application note Fluorescence polarization-based assay suitable for screening for H-Prostaglandin D Synthase inhibitors the CLARIOstar and PHERAstar FSX were used for inhibitor screening and the assay was shown to be suitable for high throughput studies. Prostaglandin D Synthase interacts intracellularly with GPCRs and serves to selectively promote GPCR expression on the cell surface. The assay comprised the Prostaglandin D Synthase (hematopoietic-type) FP-Based Inhibitor Screening Assay Kit from Cayman Chemicals which provides a convenient one-step assay. The binding of inhibitors disrupts the binding of the fluorescein-conjugated probe which decreases the fluorescence polarization signal (fig. 17).
AlphaScreen®
AlphaScreen® is a family of related technologies often used for high-throughput screening in the life sciences. Amplified Luminescent Proximity Homogeneous Assays like AlphaScreen offer bead-based chemistry that can be used to study interactions between molecules in a microplate or used as immunoassays to quantify analytes. At the heart of the AlphaScreen assay is the diffusion of singlet state oxygen from donor to acceptor beads. When a biomolecular interaction takes place that brings the donor and acceptor beads into proximity, a cascade of chemical reactions ensues that amplifies the luminescent signal in the region 520-620 nm.
In the application note An AlphaScreen SureFire® Phospho-ERK1/2 assay, ERK phosphorylation is demonstrated at receptors that signal via Gi and Gq G-proteins. The assay was performed in a 384-well format and dose-response curves for Gi and Gq agonists were determined.
The AlphaScreen SureFire Phospho-ERK1/2 assay is based on a sandwich immunoassay principle. A phosphorylated cellular protein (analyte) is sandwiched between a streptavidin-coated donor bead associated with an analyte antibody and a protein A-conjugated acceptor bead associated with an anti-phosphoprotein specific antibody (fig. 18). Phosphorylation of the analyte leads to an increase in the luminescence signal.
The body of knowledge on GPCRs and signal transduction has been built over several decades. For a long time, it was unclear how cells sensed their environment. It was thought that some type of receptor on the cell surface was involved but evidence was lacking for what this molecule might be and how it might work.7
Crucial contributions were made by many researchers across the globe including Robert J. Lefkowitz and Brian K. Kobilka who were awarded the Nobel Prize in Chemistry in 2012 for studies of GPCRs. Lefkowitz identified several receptors including the β-adrenergic receptor that binds adrenalin by radioactive labelling experiments.8,9 The receptor was eventually extracted and an initial understanding of how it worked emerged.
Kobilka joined Lefkowitz in the 1980s charged with the task of identifying the gene that encodes the β-adrenergic receptor. Analysis of the gene revealed that there was a whole family of related receptors that carried out similar functions. The studies of Lefkowitz and Kobilka were crucial for understanding how G protein-coupled receptors worked and opened up many new avenues for research and drug development.
In 2011, Kobilka and his research team published the structure of the β-adrenergic receptor at the exact moment when it is activated by a hormone and sends a signal into the cell.4 Research has continued at pace in subsequent years. The human genome revealed that at least 1000 receptors are part of the GPCR family. While many of these receptors have been studied in detail and their crystal structures are known, much remains to be discovered and the potential to find new drugs for these targets is considerable.
Over the years, BMG LABTECH microplate readers have been used in a wide range of studies that have contributed to what we know about GPCRs.
In 2018, for example, Robert Lefkowitz’s team described small molecule positive allosteric modulators of the β-adrenergic receptor through screening from DNA-encoded libraries.10 Labeling experiments for the β2-adrenergic receptor were performed on a CLARIOstar Plus microplate reader. In 2020, the CLARIOstar Plus was used by the Lefkowitz team for G protein GTPase assays as well as receptor labelling experiments in a paper published in Nature describing the structure of the M2 muscarinic receptor-β-arrestin complex.11
The number of studies on GPCRs using BMG LABTECH microplate readers continues at pace. Recently, the PHERAstar FSX was used for binding studies to identify intracellular allosteric modulators using a NanoBRET platform together with fluorescently labelled G protein peptide mimetics. The same platform offers options to probe orthosteric agonist efficacy and the dynamics of receptor activation.12
In a further study published in Proceedings of the National Academy of Sciences a CLARIOstar Plus was used as part of the screening protocol for a high-throughput CRISPR platform for multiplexed G protein-coupled receptor profiling and ligand discovery.13 These are just a few examples of how BMG LABTECH microplate readers can contribute to GPCR research.
Whatever your requirements in GPCR research, BMG LABTECH has the microplate reader for your applications.
Each BMG LABTECH multi-mode reader comes with different detection modes to match the diverse needs for the successful completion of your interaction, reporter or immunological assays. All BMG LABTECH microplate readers have exceptionally fast reading capabilities.
Collectively, BMG LABTECH multi-mode readers combine high-quality measurements with miniaturised assays, short measurement times, and offer considerable savings on materials and other resources.
Powerful and most sensitive HTS plate reader
Most flexible Plate Reader for Assay Development
Upgradeable single and multi-mode microplate reader series
Flexible microplate reader with simplified workflows
Degrons are specific sequences of amino acids or structural motifs within a protein that are important for targeted protein degradation. Find out how microplate readers can advance research into natural and engineered degrons.
Nitration can significantly modify the structure and function of proteins in cells. But how can measurements of protein nitration reveal crucial information about biological activity and how can microplate readers help?
Innovation for targeted protein degradation and next-generation degraders is gathering pace. This blog introduces some of the different approaches that act via the lysosome or proteasome.
The choice of assay for targeted protein degradation studies is crucial. But what is the preferred assay and detection technology for your specific research needs and microplate reader?
Molecular glues are small molecules that help target unwanted proteins for destruction by the ubiquitin-proteasome system. Find out how microplate readers can advance molecular glue research.
PROTACs are small, readily designed molecules that target unwanted proteins to the cell’s ubiquitin-proteasome system for degradation. Find out how microplate readers can advance PROTAC research.