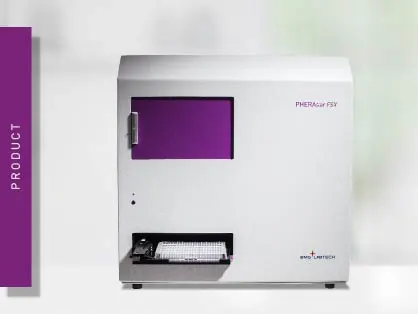
PHERAstar FSX
Powerful and most sensitive HTS plate reader
Time-Resolved FRET (TR-FRET) is a detection technology that combines Time-Resolved Fluorescence (TRF) with Förster´s Resonance Energy Transfer (FRET). TR-FRET is mainly used to analyze binding events and for high-throughput drug screening.
Förster´s Resonance Energy Transfer describes a transfer of energy between two fluorophores. It is named after the German scientist Theodor Förster who originally developed the theory of resonance energy transfer.1 For it to take place, a donor fluorophore that has absorbed energy upon excitation and finds itself in an electronically excited state, transfers energy to a second acceptor fluorophore. The transfer of energy reduces the emission intensity of the donor while increasing the acceptor’s emission intensity.
The energy transfer depends on two conditions. Firstly, the spatial proximity between donor and acceptor must be in the range of 20 - 90 Å. Resonance energy transfer is inversely proportional to the sixth power of the donor-to-acceptor distance, hence it is very sensitive to distance changes. Secondly, an overlap between the donor´s emission spectrum and the acceptor´s excitation spectrum is required. “FRET pairs” require enough distance in the emission peaks to be optically distinguishable but at the same time enough spectral overlap to allow for an efficient energy transfer (fig. 1A).
In biology, genetically encoded fluorescent proteins are most commonly used. The most popular include Green Fluorescent Protein (GFP) Red Fluorescent Protein (RFP), Cyan Fluorescent Protein (CFP), and Yellow Fluorescent Protein (YFP).2
FRET is generally used to study molecular interactions or binding events and can be employed to determine the binding affinity of two binding partners. These are assessed by linking each binding partner to be investigated to a fluorophore, and by detecting the level of energy transfer taking place (fig. 1 B, C). However, since standard FRET is negatively affected by the background noise derived from scattered excitation light and autofluorescence, highly sensitive measurement can be hardly obtained with this approach.
Time-resolved FRET eliminates the short-lived background noise derived from scattered excitation light and autofluorescence thanks to the adoption of time-resolved fluorescence and the introduction of a time-delayed detection. This is enabled by the use of lanthanides as donors. These fluorophores have large Stokes shifts and emission lifetimes up to milliseconds long.
TR-FRET relies on resonance energy transfer taking place between a lanthanide and short-lived fluorophore, when in close proximity. Because of these properties, it has improved stability and specificity over standard FRET. Buffer or medium interference is significantly reduced by the long-lived fluorescence and by the ratiometric detection of the two emission wavelengths of donor and acceptor.
The general principle of how this technology is applied is very similar throughout the different kit manufacturers, the most popular of which include HTRF®, LANCE®, LanthaScreenTM, and THUNDERTM. Donor and acceptor are either covalently bound to the interacting partners or a specific antibody against each of the two targets (or tags) is labelled with either the donor or the acceptor. By exciting the donor, energy will be transferred to the acceptor, which will emit because of fluorescence resonance energy transfer, if the targets are in close proximity. The output is proportional to the amount of binding taking place.
In TR-FRET, different fluorophores, forming different pairs are used, depending on the manufacturer.
Lanthanides are typically excited at 320 - 340 nm. The emission of europium is usually detected at 620 nm, whereas terbium can be detected at either 490 or 620 nm depending on the kit and manufacturer. For acceptors, the emission is either 520 nm for green fluorophores or 665 nm for red ones. The emission of the donor is used as an internal reference, while the acceptor emission is an indicator of the energy transfer (binding event) taking place (fig. 3).
Between excitation and fluorescence detection, a time delay in the microsecond range is applied. This eliminates the non-specific short-lived background fluorescence. Emission is then usually detected over a time period (measurement window) of microseconds (fig. 4). Depending on the kit and manufacturer, time delay and measurement window settings may differ.
A ratio of the integrated signals over time of the two emission channels is then calculated. This ratiometric measurement normalizes the signal, eliminates medium interference and quenching, and corrects for liquid handling errors or well-to-well variability.
Sensitivity and versatility made TR-FRET assays widely popular. The combination of time-resolved detection and ratiometric measurements significantly increases the assay window and reduces the background. In addition, lanthanide emission does hardly bleach, is stable over time, and is compatible with different reagents and experimental conditions.
Despite these benefits, the major advantage of this method is probably that detection of the bound pair does not require physical separation from the unbound components in order to reduce the background. As a so-called homogeneous assay, TR-FRET does not need in-between washing steps and can be run as a simple add-and-read assay (fig. 5), minimizing handling steps and being more convenient and less time-consuming than ELISAs. Consequently, it is particularly suited for automated HTS screening campaigns.
However, signal quenching generated by external interactions with the intramolecular excitation process or fluorescence of library compounds or biological proteins can be a limitation.3
TR-FRET assay kits are available from different manufacturers. Although all assays are based on common technology and can all be detected on a microplate reader, differences exist among kits and manufacturers. These are mainly related to different donor and acceptor types, combinations thereof, and different timings for detection start and measurement window.
All assay technologies and their features, including excitation and emission peaks, are summarised in table 1. Besides the outlined characteristics, these kits vary as well in their interval times and assay windows. For more details, please consult the corresponding user manuals.
Table 1: comparison of the most popular TR-FRET assay kits and their excitation and emission peaks
Kit | Donor | Cage Type | Excitation | Emission | Acceptor | Emission |
LANCE | Europium | Chelate | 320 nm | 620 nm | ULightTM | 665 nm |
LANCE | Europium | Chelate | 320 nm | 620 nm | Surelight® APC | 665 nm |
LanthaScreen Eu | Europium | Chelate | 320 nm | 620 nm | AlexaFluor 647 | 665 nm |
LanthaScreen Tb | Terbium | Chelate | 340 nm | 490 nm | Fluorescein/GFP | 520 nm |
HTRF Red (Eu) | Europium | Cryptate | 320 nm | 620 nm | XL665/d2 | 665 nm |
HTRF Red (Tb) | Terbium | Cryptate | 340 nm | 620 nm | XL665/d2 | 665 nm |
HTRF Green (Tb) | Terbium | Cryptate | 340 nm | 620 nm | Fluorescein/GFP | 520 nm |
Transcreener TR-FRET | Terbium | Chelate | 340 nm | 620 nm | HiLyte647 | 665 nm |
THUNDER | Europium | Chelate | 320 nm | 620 nm | Far-red dye | 665 nm |
Besides the use of commercially available kits, TR-FRET donor and acceptor pairs can also be combined freely, as long as the donor emission spectrum shows sufficient overlap with the acceptor’s excitation spectrum., as shown in AN 388: Differential binding of ∆9-tetrahydrocannabinol derivatives to type 1 cannabinoid receptors (CB1).
Time-resolved FRET detection is predominantly performed on microplate readers. The basic setup of a TR-FRET plate reader consists of a light source, excitation and emission filters for wavelength selection, and a photomultiplier tube (PMT) detector. Besides, the TRF detection mode, plate readers have to be capable of measuring two emission channels in one run, either sequentially or simultaneously. As TR-FRET is mainly used in high-throughput screening, compatibility with 384- and 1536-well microplates is generally compulsory.
Light sources
Chelate or cryptate complexes are typically excited at 320 - 340 nm. Therefore, both a xenon flash lamp or a specific excitation laser can be used as light source. A TRF laser focuses more energy on this specific wavelength range and leads to better results with better discrimination between low and high signals.
The PHERAstar FSX is equipped with a TRF laser with a 60 Hertz flash frequency, providing a significant speed advantage for TR-FRET detection. The laser allows the measurement of a full 1536-well plate in 36 seconds while keeping a Z´ > 0.8 for cell and biochemical assays (fig. 7), as shown in the application note “Cellular and biochemical HTRF assays measured in 1536-well microplates”.
In the application note Excellent assay performance of THUNDER™ TR-FRET cell-based cytokine assays performed on the PHERAstar FSX, it is shown how the PHERAstar FSX with TRF laser exhibits much higher sensitivity and dynamic range than a competing HTS microplate reader.
The PHERAstar FSX is equipped with photon-counting detectors (PMTs) for TR-FRET detection. Ordinary detectors provide as output an integration value for the area under the curve during the integration time. Photon-counting PMTs instead count each individual photon and thereby monitor the whole lanthanide decay curve.
On the PHERAstar FSX reader, photon-counting detection allows the measurement and display of the emission decay curve with a time resolution of 2 microseconds. This unique feature called Decay Curve Monitoring simplifies assay development and helps to optimize timing parameters, improving detection and reducing background. Together with the Integration Wizard, Decay Curve Monitoring offers a platform for TR-FRET assay optimization.
Dual-channel detection
Detection of the two emission wavelengths is required, either sequentially or simultaneously. Commonly, microplate readers measure the two emissions one after the other. Simultaneous detection however has several advantages.
The Simultaneous Dual Emission (SDE) detection system on the PHERAstar FSX uses two matched PMTs for the parallel measurement of the 2 emission wavelengths. This way, SDE reduces read times by half and increases throughput, while correcting variations caused by fill volume differences, concentrations, or fluctuations in excitation energy. These features result in improved sensitivity and lower %CVs. SDE single-channel measurements are combined for the ratiometric calculation of the results from both emission channels.
In drug screening, assay downscaling is a key step. Miniaturization aims at reducing sample volumes while maintaining reproducibility, reliability, and assay robustness. Assays can be downscaled to 3456-well plates – 384- and 1536-well formats though are most common.
TR-FRET assays can be miniaturized while maintaining accuracy and reproducibility, as signal intensity does not depend on the quantity of a tracer but on its concentration. This is further supported by the use of a highly sensitive plate reader like the PHERAstar FSX.
In the scientific talk “Successfully downscaling screening assays: Servier´s experience“, we present a case study where the PHERAstar FSX plate reader significantly contributed to the miniaturization of a HTRF assay from the 384- to the 1536-well format. On the PHERAstar FSX, miniaturization was combined with the further dilution of reagents and with “on-the-fly” detection (only one flash per well). This resulted in high-quality Z´ values and a significant reduction in reagent usage and time consumption.
TR-FRET is used to analyze binding events in biochemical assays. In addition, it is suitable for cell-based assays, since measurements of cell lysates can be performed in the presence of a culture medium (homogeneous detection).
The different biological settings include protein-DNA/RNA interactions, protein-protein (ligand-receptor) binding, kinases, and signaling pathways including GPCRs, cytokines, and biomarkers.
TR-FRET is particularly popular in the drug screening community because of its sensitivity, higher throughput, reliability and flexibility, and fewer false-positive or false-negative results.
Molecular interactions
Molecular interactions between proteins and between proteins and nucleic acids take place at every level in a cell. These include epigenetics (e.g. histone-modifying activity, where they can be used to investigate inhibition of histone deacetylases), signal transduction (e.g. G-protein activation), cellular communication (e.g. ligand-receptor interaction), gene regulation, etc. Protein-protein interactions also play a crucial role in the way PROteolysis TArgeting Chimeras (PROTACs) or molecular glues, small molecules that selectively target unwanted proteins to the cell’s protein disposal system (ubiquitin-proteasome system), function. Researchers can choose from different options for cell-based protein degrader assays. For targeted protein degradation by PROTACs and molecular glues, techniques like AlphaScreen® , Time-Resolved Fluorescence Resonance Energy Transfer (TR-FRET) or luminescence methods like NanoBRET™ can be performed in microplates to measure the interaction between target and ligase.
Kinase activity assays
Kinase activity is mainly analyzed with sandwich assays measuring the phosphorylation change of a substrate, as shown in the application note Analysis of ERK1/2, p38αβγ, and STAT3 phosphorylation with the THUNDER™ TR-FRET cellular kinase immunoassays. Competition assays can be employed as well to monitor different products generated by the reaction (e.g. ADP). Alternatively, a fluorescently labelled kinase substrate and a lanthanide labelled anti-phospho antibody is used (fig. 8), as shown in the application note “LanthaScreen TR-FRET tyrosine kinase and protein kinase C assay”. These methods can also be applied to proteases and ubiquitination. For more information on kinase activity detection visit our blog post “Kinase assays”.
GPCRs
Assays to study G-Protein Coupled Receptors (GPCRs) can either be mechanistic or functional. Functional assays focus mainly on secondary messenger quantification (e.g. IP1 or cAMP), as these may accumulate in the cell as a consequence of the activity of specific inhibitors. Accordingly, second messengers are used as a readout, since their concentration correlates with ligand binding and receptor engagement. This is shown in the application notes “HTRF IP-One assay used for functional screening” and “GPCR activation is measured using Cisbio's cAMP and IP1 HTRF HTplex cell-based assay”. Mechanistic assays focus on receptor organization and oligomerization on the cell membrane to mediate signaling.
Biomarkers
The analysis of downstream signaling products can be used to identify aberrant pathways, possibly playing a role in neurologic, metabolic, and inflammatory diseases. Time-resolved FRET can be used to test the efficacy of compounds specifically targeting these diseases. Application examples include “Development of a rapid HTRF insulin assay” for metabolic diseases, in particular diabetes mellitus, “Detection of human tau protein aggregation” in neurodegenerative diseases, and Excellent assay performance of THUNDER™ TR-FRET cell-based cytokine assays performed on the PHERAstar FSX for cytokine quantification in different diseases.
In the scientific talk Targeting the type 1 cholecystokinin receptor to screen for novel obesity treatments it is discussed how a TR-FRET assay was used to screen for the accumulation of inositol monophosphate, a downstream metabolite of IP3. Based on these results a further TR-FRET-based binding assay was developed.
Analysis of kinetic binding events
Due to technical difficulties and relatively low-throughput, the kinetics of ligand-receptor binding have been traditionally studied at a late time point in drug discovery. However, drug discovery would extremely benefit from their optimization, as Kon and Koff rates impact efficiency, the occurrence of side effects, and efficacy and duration of action. Additionally, binding kinetics seem to play a role in biased agonism. It is desirable hence to screen the binding kinetics of drug candidates at an early time point, before moving to in vivo models and clinical studies.
Binding kinetic studies (fig. 9) can be efficiently performed on plate readers that enable TR-FRET kinetic detection for screening purposes and the kinetic study of low-affinity compounds, as discussed in the scientific talk “A TR-FRET approach to measure the kinetics of ligand-receptor binding and its application of fragment screening” and in the application note “Analyze binding kinetics with HTRF”.
Its unique features dedicated to kinetic binding studies, make the PHERAstar FSX superior to any other microplate reader currently on the market. The reader can easily resolve binding events and calculate Kon and Koff rates thanks to its high temporal resolution in TR-FRET detection and dedicated hardware/software solutions.
Powerful and most sensitive HTS plate reader
Most flexible Plate Reader for Assay Development
Flexible microplate reader with simplified workflows