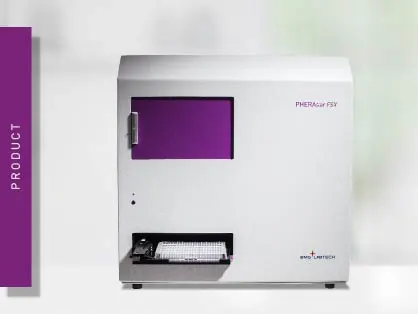
PHERAstar FSX
Powerful and most sensitive HTS plate reader
Cross-talk identifies the signal interference caused by the stray light emitted by the surrounding wells of a microplate while detecting the light signal of a specific well. In other words, cross-talk is the light from any but the well to be measured that reaches the detector and is unspecifically quantified by microplate readers.
In microplate readers, cross-talk signals from adjacent wells can reach the detector (PMT) in two different ways: either shining above the microplate to the PMT or shining through the plastic wall of a well to adjacent samples (fig. 1).
Cross-talk negatively affects assay data quality, as it can artificially increase measurement counts depending on the signal from the surrounding wells. Low-signal wells can generate more luminescence counts because of the signal cross-talk from nearby bright wells, leading to an overestimation of dimmer samples. In addition, cross-talk can increase blank variability and background noise, as well as the possibility of false positives, generally impairing data quality and statistics. Accordingly, cross-talk has to be reduced as much as possible.
On microplate readers, cross-talk specifically affects luminescence assays and “related technologies” such as AlphaScreen® and AlphaLISA® as these methods generate luminous signals with high intensity and for prolonged times. Cross-talk is typically not an issue in fluorescence intensity assays, as fluorescence emission does only occur after excitation and typically only has a very short lifetime.
Since in both luminescence and AlphaScreen/AlphaLISA assays, the light produced is diffuse, it may not only shine directly above the well but also to adjacent wells on the microplate and directly to the site of detection, while measuring another well. This leads to biased signals, higher signal variations and a lower overall sensitivity. The problem can be solved by different means, depending on the reaction type and the diffusion of the luminescent signal.
Luminescence reactions are classified into two types, flash and glow assays, based on the duration of the signal output. In short-lived flash luminescence assays, the reaction typically reaches its peak and dissolves within seconds (fig. 2, left). As the signal decays quickly, the only wells affected by cross-talk are the adjacent ones when measured directly after each other.
In contrast, glow luminescence assays develop and emit stable signals for hours (fig. 2, right). Here, cross-talk signals can reach the detector of the luminescence plate reader in two ways: shining above the plate to the detector, or through the wall of the wells. Both ways need to be approached differently.
In AlphaScreen, cross-talk is mainly caused by previously excited wells that still emit some light upon detection. A second possible cause is unwanted excitation caused by scattered light partially exciting wells on the microplate and bleaching the chemistry before the very measurement takes place.
There are multiple factors to consider when trying to reduce cross-talk. Some can be directly influenced by the user or by the assay design, others are more complex and require dedicated microplate reader solutions. Besides the nature of the assay, the two most relevant factors however are the microplate itself and the microplate reader setup.
Microplate layout
The sample distribution in the microplate should be planned carefully to avoid cross-talk as much as possible. As cross-talk is at its highest when a well with a strong signal is positioned nearby a well with low or no signal, positive controls should never be pipetted next to blanks or negative controls on a microplate. Moreover, high-signal samples should be “isolated” from the others, ideally, including empty wells between all your samples (fig. 3).
Unfortunately, it is not always possible to predict which experimental samples will give an intense or weak signal (e.g., when testing unknown compounds). In addition, sometimes you cannot afford wasting precious real estate on your microplate (e.g., high-throughput screening). As a result, it is inevitable that strong and weak samples will end up in adjacent wells on a microplate.
Microplate colour and design
White microplates are best suited for luminescence detection as they magnify the signal by reflection. Detailed information on the microplate choice can be found in our blog post: "The microplate: utility in practice." and in the HowTo Note 6: How to choose the best microplate colour for my assay.
The colour of a microplate influences cross-talk through the well walls: the darker the plate the lower the cross-talk. Measuring luminescence assays in black microplates significantly reduces cross-talk and limits background and measurement variability. However, due to overall reduced signal intensities, the assay window is also significantly impaired. Accordingly, black microplates should only be used in luminescence when very strong signals are expected. Grey microplates offer a compromise between cross-talk reduction and signal magnification.
Well-density and geometry play major roles in cross-talk reduction. The distance between wells, possible shared well walls and their thickness have a significant influence on the level of cross-talk. Higher density microplates (e.g. 1536 well) display typically higher cross-talk through the well wall than lower density microplates, as the walls are thinner. A second aspect is well geometry: square wells have a higher through-the-wall-cross-talk because of shared walls with adjacent wells. Accordingly, round, separated wells allow lower cross-talk (fig. 4).
BMG LABTECH´s PHERAstar® FSX, CLARIOstar® Plus and VANTAstarTM microplate readers offer a cross-talk reduction package that includes dedicated solutions to reduce the negative effect cross-talk can have on your data and improve data quality.
Order of well measurement
In flash luminescence assays, cross-talk can be avoided by modifying the order in which wells are measured by the microplate reader. If the microplate reader measures every second well instead of moving to the adjacent well, the cross-talk is drastically reduced by spatial and by “temporal” distance.
For this purpose, BMG LABTECH microplate readers offer an interlaced reading mode. This mode measures the first well, skips the second and jumps to the third. Every other well is measured until the end of the microplate is reached. Then, the omitted ones are measured by the microplate reader. This allows the first measured signals to decay until adjacent wells are measured (fig. 5).
Similarly, a modified reading order can be applied to AlphaScreen assays.
Physical blocking
For glow luminescence and AlphaScreen assays, microplate readers use an aperture to physically block stray light. An aperture is a black spoon-shaped accessory with a hole in the middle that is placed on top of the microplate with the hole positioned on the well to be measured. Through the hole, the light of the well of interest reaches the detector, while light coming from surrounding wells is physically blocked (fig. 6). This approach reduces the cross-talk that is generated by stray light shining from surrounding wells above the microplate to the detector.
Mathematical cross-talk reduction
White opaque microplates are not completely lightproof. Hence, light emitted by a well can shine through the plastic wall of the well and reach an adjacent sample. Light shining through the plastic walls cannot be reduced by apertures (fig. 7). As mentioned above, the type of microplate and its geometry are the most significant factors affecting this type of cross-talk.
If it is not possible to further optimise the used microplate, a mathematical cross-talk reduction can be applied to the acquired data. To this extent, the bleed-through-plastic signal to adjacent wells can be empirically determined depending on the microplate type and the sample volume used, and an algorithm can correct data upon measurement.
Applying the mathematical cross-talk reduction package helps significantly reducing signal cross-talk through the walls and increasing data quality (fig.8). However, the use of a microplate that reduces as much as possible bleed-in through the plastic walls has the largest impact on data quality.
Powerful and most sensitive HTS plate reader
Most flexible Plate Reader for Assay Development
Flexible microplate reader with simplified workflows