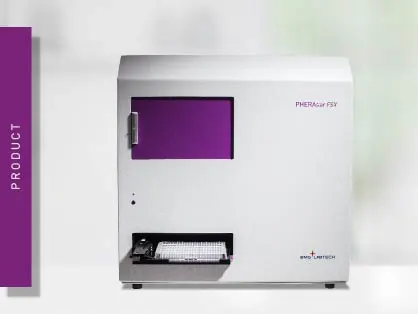
PHERAstar FSX
Powerful and most sensitive HTS plate reader
The tau protein plays a critical role in many neurological diseases and disorders. Find out about neuronal toxicity induced by tau and how microplate readers can aid tau research.
The tau protein was first discovered in the laboratory of Professor Marc Kirschner in 1975. It has attracted much interest from researchers since it was first identified as the main component of neurofibrillary tangles in the brain of patients with Alzheimer’s disease. Today the deposition of tau protein in the brain is believed to play a role in several neurodegenerative diseases and disorders that impose a significant burden on public health. Worldwide, dementia, which includes Alzheimer’s disease, is currently the seventh leading cause of death and one of the major causes of disability and dependency among older people globally (World Health Organization).
In this blog, we look at the relationship between the tau protein and the group of diseases known as tauopathies. We also discuss how microplate readers can be used in neuroscience to accelerate research into the naturally occurring tau protein.
In a healthy brain, the tau protein is an extended molecule that consists of 352-441 amino acids. In diseases like Alzheimer’s, tau can become abnormally folded and form aggregates called neurofibrillary tangles (fig. 1). These tangles are a hallmark of several neurodegenerative diseases and disorders including Alzheimer’s and are thought to contribute to neuronal dysfunction and cell death.
Six known isoforms of the tau protein exist, and the number of amino acids in each depends on the specific isoform. Each tau comprises a series of repeated sequences called microtubule-binding domains interspersed with flexible linker regions (fig. 2). The microtubule-binding domain consists of a series of three or four repeats of a 31-34 amino acid motif, which folds into a beta sheet structure. These domains are the structural fabric of the tau protein that interact with and stabilize microtubules. Microtubules are a crucial part of the cytoskeleton that maintain cell shape and provide tracks for intracellular transport. The linker regions between the microtubule-binding domains are flexible structures that permit the protein to adopt different conformations.
Tau is encoded by a single gene, but multiple splicing of the pre-mRNA sequences produces different isoforms of the protein. Alternative splicing of exons 2, 3 and 10 generates the six possible isoforms. Each isoform has different physiological functions and likely different roles in Alzheimer’s disease, frontotemporal dementia, and related disorders. Some isoforms are more prone to aggregation, others are commonly found in tangles.
The physiological role of the tau protein in neurons is to stabilize microtubules and regulate their dynamics. This is essential for the structural integrity and proper function of the neuron. Crucially, healthy tau binds to microtubules in the neuron and promotes the assembly of microtubules and their stability. This maintains the overall structural integrity of the neuron and ensures proper movement of proteins, organelles and vesicles along the length of the axon.
Tau specifically interacts with motor proteins like kinesin and dynein, which move along microtubules and transport cargo along the axon. In this context, the tau protein helps control the speed and direction of axonal transport by regulating the attachment and detachment of these motor proteins to microtubules.
When tau accumulates in the form of abnormal neurofibrillary tangles, it is likely a footprint to disease. However, direct causation of the actions of the tau protein with full-blown diseases like Alzheimer’s and frontotemporal dementia remains to be fully established.
Tau can lose its function in several ways, and it is likely that multiple mechanisms are involved in the development of neurodegeneration and diseases like Alzheimer’s.1,2
Hyperphosphorylation, where tau becomes phosphorylated at many more sites than normal by kinase enzymes, can cause the tau protein to become detached from microtubules. This interrupts the stability mentioned earlier and leads to destabilization and neuronal dysfunction.
Tau can also become aggregated forming clumps or oligomers in the cytoplasm of neurons. It is thought that this type of aggregation can be triggered by events like oxidative stress, inflammation, or genetic mutations. Tau aggregates interfere with cellular processes and may aggregate further to form neurofibrillary tangles. The accumulation of abnormal tangles is thought to lead to the death of neurons, neurodegeneration and eventually diseases like Alzheimer’s.
An imbalance of the different isoforms of the tau protein is also a potential trigger for dysfunction. For example, an imbalance in the 3R:4R isoform ratio (where R represents a microtubule-binding repeat) has been implicated in certain types of frontotemporal disorders but the underlying mechanisms still need to be established.
Since the tau protein interacts with many other proteins in the cell some of these interactions may be involved in dysfunction and neurodegeneration. For example, protein-protein interactions between tau and amyloid-beta protein may lead to the formation of abnormal toxic aggregates that contribute to Alzheimer’s disease, but further work is needed to confirm a causal link due to these specific interactions.
The dysfunction of tau can involve multiple pathways that include kinase activation, impaired protein clearance (for example by the ubiquitin-proteasome system and autophagy pathways), oxidative stress, inflammation, and the dysfunction of certain chaperone proteins. Chaperone proteins help maintain protein folding and prevent the aggregation of misfolded proteins. Dysfunction of chaperones may lead to the accumulation of misfolded tau proteins, which promotes the formation of toxic aggregates.
Tau has also been implicated in synaptic dysfunction, the communication or signaling process that occurs between neurons. Hyperphosphorylation or aggregation of the tau protein is thought to be involved in the disruption of synaptic function which may impair axonal transport and the delivery of synaptic proteins and organelles to the synapse. In some cases, the tau protein may modify synaptic proteins by affecting their expression or localization.
Calcium is essential for healthy synaptic function. The excessive influx of calcium into neurons can lead to the activation of enzymes such as kinases or proteases which can promote the aggregation or hyperphosphorylation of tau. This in turn may lead to synaptic dysfunction or loss of synapses.
Another process that may be affected by tau is the pruning of synapses. Synaptic pruning is a naturally occurring process regulated by microglia, a type of glial cell located throughout the brain and spinal cord. Microglia make up 10-15% of brain cells and represent the front line of immune defense in the central nervous system. In vitro experiments with mouse microglial cells combined with in vivo studies in a mouse model system have shown that the tau protein directly activates the NF-κB pathway in microglia and triggers an inflammatory process.3 This in turn may impact synaptic pruning.
The collective family of tau neurodegenerative disorders are known as tauopathies.4 Tauopathies are defined as neurodegenerative disorders that involve the deposition of the tau protein in the brain. As a group of pathologies, tauopathies extend beyond traditional diseases like Alzheimer’s, corticobasal degeneration and progressive supranuclear palsy to include emerging pathologies like globular glial tauopathies, neurofibrillary tangle dementia and age-related tauopathies. 5 Clinical symptoms of tauopathies include frontotemporal dementia, parkinsonism, akinesia, motor neuron symptoms or cerebellar ataxia. The role of the tau protein in these different conditions hinges on many cellular processes and molecular events and much remains to be established as to how tau contributes to each disorder.
Different therapeutic approaches target the tau protein. However, while some of these approaches are in clinical trials no treatments specifically for tau-related disease have received regulatory approval. What type of therapeutic approaches are being pursued for Alzheimer’s and other diseases in the context of tau?
Researchers are looking for inhibitors that prevent the aggregation of tau or promote its clearance. Since tau is hyperphosphorylated in several disorders and diseases, including Alzheimer’s, kinase inhibitors are also being explored. Beyond small molecule inhibitors, attention is also turning in the long term to immunotherapies and gene therapy as potential treatment options.
Immunotherapies are designed to stimulate the immune system to recognize and remove tau aggregates or to deliver specific antibodies that bind to tau. Gene therapy approaches span the delivery of genes that can reduce tau expression, promote tau clearance, or target specific forms of aggregation. For example, researchers are trying to treat chronic traumatic encephalopathy, a progressive neurodegenerative disorder, using an approach that involves anti-phospho-tau gene therapy.6 Administration of an adeno-associated virus vector coding for an anti-pTau antibody may generate sufficient levels of antibody to suppress phosphorylated Tau (pTau) accumulation and put the brakes on the disease process. This type of research is at a very early stage.
Other approaches for diseases like Alzheimer’s include finding therapeutics that may help to stabilize microtubules to counteract the effects of tau pathology. Drugs like taxanes come in this category but are also at early stages of clinical investigation. Since neuroinflammation is a feature of several neurological disorders, researchers are also looking to find drugs that target the inflammatory response. This in turn may help to reduce any potential pathological effects of tau.
Microplate readers offer high-throughput capabilities and a wide range of applications relevant to the study of Alzheimer’s and other diseases.
For example, they are ideally suited to detect and quantify the phosphorylation status of tau proteins using specific antibodies that recognize phosphorylated tau. Many of these approaches involve enzyme-linked immunosorbent assays.
In the application note Comparing site-specific phospho-tau in normal and Alzheimer’s disease brains, an ELISA assay was used to detect two phospho-tau modifications at threonine 181 and threonine 217. Both modifications have been proposed as functional diagnostic biomarkers to distinguish between a healthy state and Alzheimer’s disease. In these experiments, both tau phosphorylation sites functionally distinguished normal from disease tissue with Thr217 being somewhat better than Thr181 as a potential biomarker for Alzheimer’s disease. The absorbance measurements and high dynamic range luminescence measurements on the CLARIOstar® Plus ensured the optimal performance of the ELISA kits used in the analysis (fig. 3 and fig. 4).
Microplate readers can also be used to detect tau aggregates. The application note Detection of human tau protein aggregation describes the use of Homogeneous Time Resolved Fluorescence on the PHERAstar® FSX to detect and quantify tau aggregates in brain tissue and look at the kinetics of tau aggregation and dissociation. Tau aggregates were measured using a sandwich immunoassay and a labeled anti-tau monoclonal antibody ensured reproducibility of the assay and quality of the signal. The specific HTRF signal generated is proportional to the amount of tau aggregates (fig. 5).
Moreover, the assay can clearly distinguish between samples containing chemically aggregated tau compared to non-aggregated tau (fig. 6). The use of Homogeneous Time Resolved Fluorescence enables measurement of the sample several times without the effect of bleaching. This property of the HTRF reagents allows for kinetic measurements to monitor tau aggregation in real time.
In the application note Fast and accurate detection of Alzheimer’s Disease targets with SimpleStep ELISA® kits and SPECTROstar® Nano it was possible to quantify not only tau but two other targets implicated in the progress of Alzheimer’s disease, namely Brain Derived Neurotrophic Factor (BDNF) and Triggering Receptor Expressed in Myeloid Cells 2 (TREM2). The SimpleStep ELISA kits quantify BDNF, Tau and TREM2 in a one-wash 90-minute protocol. Absorbance is measured on the microplate reader after the wash step and color development. The assay principle for the SimpleStep ELISA kits is shown in Fig. 7. Representative data for human tau are also shown (fig. 8). These kits provide fast sample analysis with little hands-on time required during the assay.
Microplate readers can also be used to screen for tau inhibitors and potential drug candidates that target tau and which may be relevant for diseases like Alzheimer’s. This may involve setting up assays that measure the activity of a tau kinase or the aggregation of tau. Aggregation can be measured using specific molecules like the fluorescent dye thioflavin T. Thioflavin T assays also have applications in prion research. More information is available in the BMG LABTECH blog Prion research.
What is the preferred BMG LABTECH microplate reader for specific needs and applications related to tau research and the study of diseases like Alzheimer’s? BMG LABTECH offers a range of detection devices for sensitive absorbance and fluorescence measurements.
The PHERAstar FSX was specifically conceived for screening campaigns and is your go-to reader for high-performance high-throughput investigations.
Both the VANTAstar® and CLARIOstar® Plus allow for emission scanning and include Enhanced Dynamic Range technology for superior performance in a single run. They also offer increased light transmission and sensitivity courtesy of Linear Variable Filter MonochromatorsTM and different filter options.
All BMG LABTECH microplate readers have exceptionally fast reading capabilities. In addition, the Omega series, CLARIOstar Plus and PHERAstar FSX microplate readers come with on-board injectors that can offer the very best options for detection at the time of injection.
BMG LABTECH’s Omega series of readers are a preferred choice for RT-QuIC aggregation assays as they have the robustness to withstand extensive and prolonged shaking.7
Collectively, these multi-mode readers combine high performance with miniaturized assays, short measurement times, and offer considerable savings on materials and other resources.
Powerful and most sensitive HTS plate reader
Most flexible Plate Reader for Assay Development
Upgradeable single and multi-mode microplate reader series
Flexible microplate reader with simplified workflows
Neurodegenerative disease ultimately leads to the death of neurons as neuronal functions deteriorate. Find out how microplate readers can be used to study neuronal cell death and its link to neurodegenerative disease.
The disruption of mitochondrial function is linked to neurodegenerative diseases. Find out how microplate readers advance research into mitochondrial dysfunction and neurodegenerative diseases.
The way proteins misfold and aggregate is linked to many neurodegenerative diseases. Find out how microplate readers can help advance research into protein misfolding.
Alpha-synuclein is a key protein involved in neurodegenerative diseases like Parkinson’s. Find out how microplate readers can help advance alpha-synuclein research.
Amyloids are thought to play a crucial role in neurodegeneration. Find out how microplate readers help advance amyloid research.